Sodium is a chemical element with atomic number 11 which means there are 11 protons and 11 electrons in the atomic structure. Since 1s can only hold two electrons the next 2 electrons for sodium go in the 2s orbital. The nex six electrons will go in the 2p orbital. The p orbital can hold up to six electrons. We'll put six in the 2p orbital and then put the remaining electron in the 3s. Therefore the sodium.
- Chemical properties
Our editors will review what you’ve submitted and determine whether to revise the article.
Join Britannica's Publishing Partner Program and our community of experts to gain a global audience for your work!Sodium (Na), chemical element of the alkali metalgroup (Group 1 [Ia]) of the periodic table. Sodium is a very soft silvery-white metal. Sodium is the most common alkali metal and the sixth most abundant element on Earth, comprising 2.8 percent of Earth’s crust. It occurs abundantly in nature in compounds, especially common salt—sodium chloride (NaCl)—which forms the mineral halite and constitutes about 80 percent of the dissolved constituents of seawater.
atomic number | 11 |
---|---|
atomic weight | 22.9898 |
melting point | 97.81 °C (208 °F) |
boiling point | 882.9 °C (1,621 °F) |
specific gravity | 0.971 (20 °C) |
oxidation states | +1, −1 (rare) |
electron configuration | 2-8-1 or 1s22s22p63s1 |
Properties and production
Because sodium is extremely reactive, it never occurs in the free state in Earth’s crust. In 1807 Sir Humphry Davy became the first to prepare sodium in its elemental form, applying electrolysis to fused sodium hydroxide (NaOH). Sodium is an important constituent of a number of silicate materials, such as feldspars and micas. There are huge deposits of rock salt in various parts of the world, and sodium nitrate deposits exist in Chile and Peru. The sodium content of the sea is approximately 1.05 percent, corresponding to a concentration of approximately 3 percent of sodium halides. Sodium has been identified in both the atomic and ionic forms in the spectra of stars, including the Sun, and the interstellar medium. Analysis of meteorites indicates that the silicate material present has an average content of approximately 4.6 atoms of sodium for every 100 atoms of silicon.
Lighter than water, sodium can be cut with a knife at room temperature but is brittle at low temperatures. It conducts heat and electricity easily and exhibits the photoelectric effect (emission of electrons when exposed to light) to a marked degree.
Sodium is by far the most commercially important alkali metal. Most processes for the production of sodium involve the electrolysis of molten sodium chloride. Inexpensive and available in tank-car quantities, the element is used to produce gasoline additives, polymers such as nylon and syntheticrubber, pharmaceuticals, and a number of metals such as tantalum, titanium, and silicon. It is also widely used as a heat exchanger and in sodium-vapour lamps. The yellow colour of the sodium-vapour lamp and the sodium flame (the basis of an analytical test for sodium) is identified with two prominent lines in the yellow portion of the light spectrum.
Significant uses
Two of the earliest uses of metallic sodium were in the manufacture of sodium cyanide and sodium peroxide. Significant quantities were used in the manufacture of tetraethyl lead as a gasoline additive, a market that disappeared with the advent of unleaded gasoline. Substantial amounts of sodium are used in the manufacture of sodium alkyl sulfates as the principal ingredient in synthetic detergents.
Sodium also is used as a starting material in the manufacture of sodium hydride (NaH) and sodium borohydride (NaBH4). In addition, sodium is employed in the production of dyes and dye intermediates, in the synthesis of perfumes, and in a wide variety of organic reductions. It is used in the purification of hydrocarbons and in the polymerization of unsaturated hydrocarbons. In many organic applications, sodium is used in the form of dispersions in hydrocarbon liquid media.
Molten sodium is an excellent heat-transfer fluid, and, because of this property, it has found use as coolant in liquid-metal fast breeder reactors. Sodium is used extensively in metallurgy as a deoxidant and as a reducing agent for the preparation of calcium, zirconium, titanium, and other transition metals. Commercial production of titanium involves reduction of titanium tetrachloride (TiCl4) with sodium. The products are metallic Ti and NaCl.
Principal compounds
Sodium is highly reactive, forming a wide variety of compounds with nearly all inorganic and organic anions (negatively charged ions). It normally has an oxidation state of +1, and its single valence electron is lost with great ease, yielding the colourless sodium cation (Na+). Compounds that contain the sodium anion, Na−, have also been synthesized. The principal commercial sodium compounds are the chloride, carbonate, and sulfate.
The most important and familiar sodium compound is sodium chloride, or common salt, NaCl. Most other sodium compounds are prepared either directly or indirectly from sodium chloride, which occurs in seawater, in natural brines, and as rock salt. Large quantities of sodium chloride are employed in the production of other heavy (industrial) chemicals as well as being used directly for ice and snow removal, for water conditioning, and in food.
Other major commercial applications of sodium chloride include its use in the manufacture of chlorine and sodium hydroxide by electrolytic decomposition and in the production of sodium carbonate (Na2CO3) by the Solvay process. The electrolysis of aqueous sodium chloride produces sodium hypochlorite, NaOCl, a compound of sodium, oxygen, and chlorine used in large quantities in household chlorine bleach. Sodium hypochlorite is also utilized as an industrial bleach for paper pulp and textiles, for chlorination of water, and in certain medicinal preparations as an antiseptic and a fungicide. It is an unstable compound known only in aqueous solution.
The carbonates contain the carbonate ion (CO32–). Sodium bicarbonate, also called sodium hydrogen carbonate, or bicarbonate of soda, NaHCO3, is a source of carbon dioxide and so is used as an ingredient in baking powders, in effervescent salts and beverages, and as the main constituent of dry-chemical fire extinguishers. Its slight alkalinity makes it useful in treating gastric or urinary hyperacidity and acidosis. It is also employed in certain industrial processes, as in tanning and the preparation of wool. Sodium carbonate, or soda ash, Na2CO3, is widely distributed in nature, occurring as constituents of mineral waters and as the solid minerals natron, trona, and thermonatrite. Large quantities of this alkaline salt are used in making glass, detergents, and cleansers. Sodium carbonate is treated with carbon dioxide to produce sodium bicarbonate. The monohydrate form of sodium carbonate, Na2CO3·H2O, is employed extensively in photography as a constituent in developers.
Sodium sulfate, Na2SO4, is a white crystalline solid or powder employed in the manufacture of kraft paper, paperboard, glass, and detergents and as a raw material for the production of various chemicals. It is obtained either from deposits of the sodium sulfate minerals mirabilite and thenardite or synthetically by the treatment of sodium chloride with sulfuric acid. The crystallized product is a hydrate, Na2SO4·10H2O, commonly known as Glauber’s salt. Sodium thiosulfate (sodium hyposulfite), Na2S2O3, is used by photographers to fix developed negatives and prints; it acts by dissolving the part of the silver salts coated onto film which remain unchanged by exposure to light.
Sodium hydroxide (NaOH) is a corrosive white crystalline solid that readily absorbs moisture until it dissolves. Commonly called caustic soda, or lye, sodium hydroxide is the most widely used industrial alkali. It is highly corrosive to animal and vegetable tissue. The alkaline solutions it forms when dissolved in water neutralize acids in various commercial processes: in petroleum refining, it removes sulfuric and organic acids; in soapmaking, it reacts with fatty acids. Solutions of NaOH are used in the treatment of cellulose and in the manufacture of many chemicals.
Sodium nitrate, or soda nitre, NaNO3, is commonly called Chile saltpetre, after its mineral deposits in northern Chile, the principal source. Sodium nitrate is used as a nitrogenous fertilizer and as a component of dynamite.
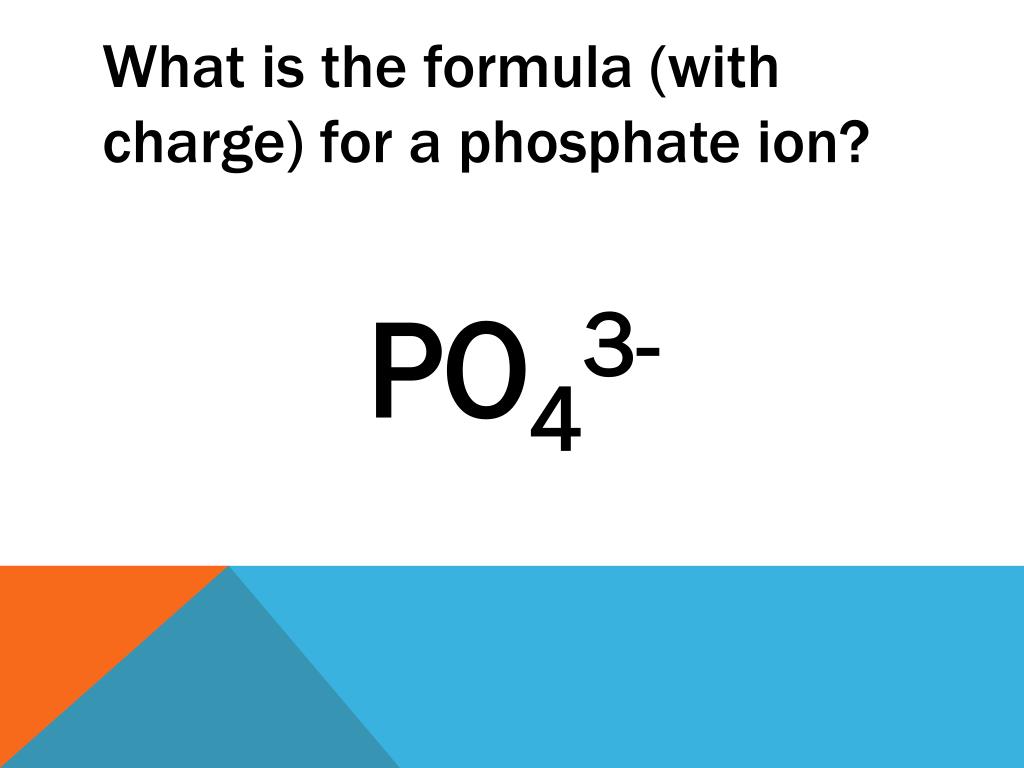
- key people
- related topics
A solvated electron is a freeelectron in (solvated in) a solution, and is the smallest possible anion. Solvated electrons occur widely, although it is difficult to observe them directly because their lifetimes are so short.[1] The deep color of solutions of alkali metals in liquid ammonia arises from the presence of solvated electrons: blue when dilute and copper-colored when more concentrated (> 3 molar).[2] Classically, discussions of solvated electrons focus on their solutions in ammonia, which are stable for days, but solvated electrons also occur in water and other solvents – in fact, in any solvent that mediates outer-sphere electron transfer. The real hydration energy of the solvated electron can be estimated by using the hydration energy of a proton in water combined with kinetic data from pulse radiolysis experiments. The solvated electron forms an acid–base pair with atomic hydrogen.
The solvated electron is responsible for a great deal of radiation chemistry.
Alkali metals dissolve in liquid ammonia giving deep blue solutions, which conduct electricity. The blue colour of the solution is due to ammoniated electrons, which absorb energy in the visible region of light. Alkali metals also dissolve in some small primary amines, such as methylamine and ethylamine[3] and hexamethylphosphoramide, forming blue solutions. Solvated electron solutions of the alkaline earth metals magnesium, calcium, strontium and barium in ethylenediamine have been used to intercalate graphite with these metals.[4]
History[edit]
Sodium Electrons Number
The observation of the color of metal-electride solutions is generally attributed to Humphry Davy. In 1807–1809, he examined the addition of grains of potassium to gaseous ammonia (liquefaction of ammonia was invented in 1823). James Ballantyne Hannay and J. Hogarth repeated the experiments with sodium in 1879–1880. W. Weyl in 1844 and C. A. Seely in 1871 used liquid ammonia while Hamilton Cady in 1897 related the ionizing properties of ammonia to that of water. Charles A. Kraus measured the electrical conductance of metal ammonia solutions and in 1907 attributed it to the electrons liberated from the metal.[5][6] In 1918, G. E. Gibson and W. L. Argo introduced the solvated electron concept.[7] They noted based on absorption spectra that different metals and different solvents (methylamine, ethylamine) produce the same blue color, attributed to a common species, the solvated electron. In the 1970s, solid salts containing electrons as the anion were characterized.[8]
Properties[edit]
Focusing on solutions in ammonia, liquid ammonia will dissolve all of the alkali metals and other electropositive metals such as Ca,[9]Sr, Ba, Eu, and Yb (also Mg using an electrolytic process[10]), giving characteristic blue solutions.
A lithium–ammonia solution at −60 °C is saturated at about 15 mol% metal (MPM). When the concentration is increased in this range electrical conductivity increases from 10−2 to 104ohm−1cm−1 (larger than liquid mercury). At around 8 MPM, a 'transition to the metallic state' (TMS) takes place (also called a 'metal-to-nonmetal transition' (MNMT)). At 4 MPM a liquid-liquid phase separation takes place: the less dense gold-colored phase becomes immiscible from a denser blue phase. Above 8 MPM the solution is bronze/gold-colored. In the same concentration range the overall density decreases by 30%.
Dilute solutions are paramagnetic and at around 0.5 MPM all electrons are paired up and the solution becomes diamagnetic. Several models exist to describe the spin-paired species: as an ion trimer; as an ion-triple—a cluster of two single-electron solvated-electron species in association with a cation; or as a cluster of two solvated electrons and two solvated cations.
Solvated electrons produced by dissolution of reducing metals in ammonia and amines are the anions of salts called electrides. Such salts can be isolated by the addition of macrocyclicligands such as crown ether and cryptands. These ligands bind strongly the cations and prevent their re-reduction by the electron.
In neutral of partially-oxidized metal-ammonia or metal-aqua complexes diffuse solvated electrons are present. These species are recognized as 'Solvated electron precursors' (SEPs). Simply a SEP is a metal complex that bear diffuse electrons in the periphery of the ligands.[11] The diffuse solvated electron cloud occupies a quasi-spherical atomic s-type orbital and populate higher angular momentum p-, d-, f-, g-type orbitals in excited states.[12][13][14][15]
Its standard electrode potential value is -2.77 V.[16] Equivalent conductivity 177 Mho cm2 is similar to that of hydroxide ion. This value of equivalent conductivity corresponds to a diffusivity of 4,75*10−5 cm2s−1.[17]
Some thermodynamic properties of the solvated electron have been investigated by Joshua Jortner and Richard M. Noyes (1966)[18]
Alkaline aqueous solutions above pH = 9.6 regenerate the hydrated electron through the reaction of hydrated atomic hydrogen with hydroxide ion giving water beside hydrated electrons.
Below pH = 9.6 the hydrated electron reacts with the hydronium ion giving atomic hydrogen, which in turn can react with the hydrated electron giving hydroxide ion and usual molecular hydrogen H2.
The properties of solvated electron can be investigated using the rotating ring-disk electrode.
Reactivity and applications[edit]
The solvated electron reacts with oxygen to form a superoxideradical (O2.−).[19] With nitrous oxide, solvated electrons react to form hydroxyl radicals (HO.).[20] The solvated electrons can be scavenged from both aqueous and organic systems with nitrobenzene or sulfur hexafluoride[citation needed].
A common use of sodium dissolved in liquid ammonia is the Birch reduction. Other reactions where sodium is used as a reducing agent also are assumed to involve solvated electrons, e.g. the use of sodium in ethanol as in the Bouveault–Blanc reduction.
Solvated electrons are involved in the reaction of sodium metal with water.[21] Two solvated electrons combine to form molecular hydrogen and hydroxide ion.
Solvated electrons are also involved in electrode processes.[22]
Hydrogen Ions React With Sodium Electrons
Diffusion[edit]
The diffusivity of the solvated electron in liquid ammonia can be determined using potential-step chronoamperometry.[23]
In gas phase and upper atmosphere of Earth[edit]
Solvated electrons can be found even in the gas phase. This implies their possible existence in the upper atmosphere of Earth and involvement in nucleation and aerosol formation.[24]
References[edit]
- ^Schindewolf, U. (1968). 'Formation and Properties of Solvated Electrons'. Angewandte Chemie International Edition in English. 7 (3): 190–203. doi:10.1002/anie.196801901.
- ^Cotton, F. A.; Wilkinson, G. (1972). Advanced Inorganic Chemistry. John Wiley and Sons Inc. ISBN978-0-471-17560-5.
- ^Greenwood, Norman N.; Earnshaw, Alan (1997). Chemistry of the Elements (2nd ed.). Butterworth-Heinemann. ISBN978-0-08-037941-8.
- ^W. Xu and M. M. Lerner, 'A New and Facile Route Using Electride Solutions To Intercalate Alkaline Earth Ions into Graphite', Chem. Mater. 2018, 30, 19, 6930–6935. https://doi.org/10.1021/acs.chemmater.8b03421
- ^Kraus, Charles A. (1907). 'Solutions of Metals in Non-Metallic Solvents; I. General Properties of Solutions of Metals in Liquid Ammonia'. J. Am. Chem. Soc.29 (11): 1557–1571. doi:10.1021/ja01965a003.
- ^Zurek, Eva (2009). 'A Molecular Perspective on Lithium–Ammonia Solutions'. Angew. Chem. Int. Ed.48 (44): 8198–8232. doi:10.1002/anie.200900373. PMID19821473.
- ^Gibson, G. E.; Argo, W. L. (1918). 'The Absorption Spectra of the Blue Solutions of Certain Alkali and Alkaline Earth Metals in Liquid Ammonia and Methylamine'. J. Am. Chem. Soc.40 (9): 1327–1361. doi:10.1021/ja02242a003.
- ^Dye, J. L. (2003). 'Electrons as Anions'. Science. 301 (5633): 607–608. doi:10.1126/science.1088103. PMID12893933.
- ^Edwin M. Kaiser (2001). 'Calcium-Ammonia'. Calcium–Ammonia. Encyclopedia of Reagents for Organic Synthesis. doi:10.1002/047084289X.rc003. ISBN978-0471936237.
- ^Combellas, C; Kanoufi, F; Thiébault, A (2001). 'Solutions of solvated electrons in liquid ammonia'. Journal of Electroanalytical Chemistry. 499: 144–151. doi:10.1016/S0022-0728(00)00504-0.
- ^Ariyarathna, Isuru R.; Pawłowski, Filip; Ortiz, Joseph Vincent; Miliordos, Evangelos (2018). 'Molecules mimicking atoms: monomers and dimers of alkali metal solvated electron precursors'. Physical Chemistry Chemical Physics. 20 (37): 24186–24191. doi:10.1039/c8cp05497e.
- ^Ariyarathna, Isuru R.; Khan, Shahriar N.; Pawłowski, Filip; Ortiz, Joseph Vincent; Miliordos, Evangelos (4 January 2018). 'Aufbau Rules for Solvated Electron Precursors: Be(NH 3 ) 4 0,± Complexes and Beyond'. The Journal of Physical Chemistry Letters. 9 (1): 84–88. doi:10.1021/acs.jpclett.7b03000.
- ^Ariyarathna, Isuru R.; Miliordos, Evangelos (2019). 'Superatomic nature of alkaline earth metal–water complexes: the cases of Be(H 2 O)0,+4 and Mg(H 2 O)0,+6'. Physical Chemistry Chemical Physics. 21 (28): 15861–15870. doi:10.1039/c9cp01897b.
- ^Ariyarathna, Isuru R.; Miliordos, Evangelos (2020). 'Geometric and electronic structure analysis of calcium water complexes with one and two solvation shells'. Physical Chemistry Chemical Physics. 22 (39): 22426–22435. doi:10.1039/d0cp04309e.
- ^Ariyarathna, Isuru (1 March 2021). 'First Principle Studies on Ground and Excited Electronic States: Chemical Bonding in Main-Group Molecules, Molecular Systems with Diffuse Electrons, and Water Activation using Transition Metal Monoxides'.
- ^Baxendale, J. H. (1964), Radiation Res. Suppl., 114 and 139
- ^Hart, Edwin J. (1969). 'The Hydrated Electron'. Survey of Progress in Chemistry. 5: 129–184. doi:10.1016/B978-0-12-395706-1.50010-8. ISBN9780123957061.
- ^Jortner, Joshua; Noyes, Richard M. (1966). 'Some Thermodynamic Properties of the Hydrated Electron'. The Journal of Physical Chemistry. 70 (3): 770–774. doi:10.1021/j100875a026.
- ^Hayyan, Maan; Hashim, Mohd Ali; Alnashef, Inas M. (2016). 'Superoxide Ion: Generation and Chemical Implications'. Chemical Reviews. 116 (5): 3029–3085. doi:10.1021/acs.chemrev.5b00407. PMID26875845.
- ^Janata, Eberhard; Schuler, Robert H. (1982). 'Rate constant for scavenging eaq- in nitrous oxide-saturated solutions'. The Journal of Physical Chemistry. 86 (11): 2078–2084. doi:10.1021/j100208a035.
- ^Walker, D.C. (1966). 'Production of hydrated electron'. Canadian Journal of Chemistry. 44 (18): 2226–. doi:10.1139/v66-336.
- ^B. E. Conway, D. J. MacKinnon, J. Phys. Chem., 74, 3663, 1970
- ^Harima, Yutaka; Aoyagui, Shigeru (1980). 'The diffusion coefficient of solvated electrons in liquid ammonia'. Journal of Electroanalytical Chemistry and Interfacial Electrochemistry. 109 (1–3): 167–177. doi:10.1016/S0022-0728(80)80115-X.
- ^F. Arnold, Nature 294, 732-733, (1981)
Further reading[edit]

- Sagar, D. M.; Colin; Bain, D.; Verlet, Jan R. R. (2010). 'Hydrated Electrons at the Water/Air Interface'. J. Am. Chem. Soc. 132 (20): 6917–6919. doi:10.1021/ja101176r. PMID20433171.
- Martyna, Glenn (1993). 'Electronic states in metal-ammonia solutions'. Physical Review Letters. 71 (2): 267–270. Bibcode:1993PhRvL..71..267D. doi:10.1103/physrevlett.71.267. PMID10054906.
- Martyna, Glenn (1993). 'Quantum simulation studies of singlet and triplet bipolarons in liquid ammonia'. Journal of Chemical Physics. 98 (1): 555–563. Bibcode:1993JChPh..98..555M. doi:10.1063/1.464650.
- Solvated Electron. Advances in Chemistry. 50. 1965. doi:10.1021/ba-1965-0050. ISBN978-0-8412-0051-7.
- Anbar, Michael (1965). 'Reactions of the Hydrated Electron'. Solvated Electron. Advances in Chemistry. 50. pp. 55–81. doi:10.1021/ba-1965-0050.ch006. ISBN978-0-8412-0051-7.
- Abel, B.; Buck, U.; Sobolewski, A. L.; Domcke, W. (2012). 'On the nature and signatures of the solvated electron in water'. Phys. Chem. Chem. Phys. 14 (1): 22–34. Bibcode:2012PCCP...14...22A. doi:10.1039/C1CP21803D. PMID22075842.
- Harima, Y.; Aoyagui, S. (1981). 'Determination of the chemical solvation energy of the solvated electron'. Journal of Electroanalytical Chemistry and Interfacial Electrochemistry. 129 (1–2): 349–352. doi:10.1016/S0022-0728(81)80027-7.
- Hart, Edwin J. (1969). 'The Hydrated Electron'. Survey of Progress in Chemistry Volume 5. Survey of Progress in Chemistry. 5. pp. 129–184. doi:10.1016/B978-0-12-395706-1.50010-8. ISBN9780123957061.
- The electrochemistry of the solvated electron. Technische Universiteit Eindhoven.
- IAEA On the Electrolytic Generation of Hydrated Electron.
- Fundamentals of Radiation Chemistry, chapter 6, p. 145–198, Academic Press, 1999.
- Tables of bimolecular rate constants of hydrated electrons, hydrogen atoms and hydroxyl radicals with inorganic and organic compounds International Journal of Applied Radiation and Isotopes Anbar, Neta
